A polypeptide is a compound formed by a plurality of amino acids linked by peptide bonds, and is ubiquitously present in living organisms. To date, tens of thousands of polypeptides have been found in living organisms. Polypeptides play an important role in regulating the functional activities of various systems, organs, tissues and cells of the body as well as in life activities, and are often used in functional analysis, antibody research, drug development and other fields. With the maturity of biotechnology and peptide synthesis technologies, more and more peptide drugs have been developed and used in clinical practice.
There are many kinds of peptide modifications, which can be easily divided into post-modification and process modification (using derivatized amino acid modification). From the different modification sites, they can be divided into N-terminal modification, C-terminal modification, side chain modification, amino acid modification, skeleton modification, etc. (figure 1). As an important means to change the main chain structure or side chain group of the peptide chain, the polypeptide modification can effectively change the physical and chemical properties of the peptide compound, increase the water solubility, prolong the time of action in vivo, change its biodistribution, and eliminate immunogenicity. Reduce toxic side effects, etc. This article focuses on several of the most important peptide modification strategies and characteristics.
1, circularization
Cyclic peptides have many applications in biomedicine, and many biologically active natural polypeptides are cyclic polypeptides. Since cyclic peptides tend to be more rigid than linear peptides, they are extremely resistant to the digestive system, can survive in the digestive tract, and exhibit a stronger affinity for target receptors. Cyclization is the most direct route to the synthesis of cyclic polypeptides, especially for polypeptides with a large structural backbone. According to the cyclization method, it can be divided into a side chain-side chain type, a terminal-side chain type, and a terminal-terminal type (head-to-tail type).
(1) sidechain-to-side chain
The most common type of side chain-side chain cyclization is the disulfide bridge between cysteine ​​residues, which is introduced by deprotection of a pair of cysteine ​​residues followed by oxidation to form a disulfide bond. Polycyclic synthesis can be achieved by selective removal of the thiol protecting group. The cyclization can be accomplished either in the solvent after dissociation or on the resin prior to dissociation. Since the polypeptide on the resin does not readily form a cyclizable conformation, cyclization on the resin may be less efficient than cyclization in a solvent. Another type of side chain-side chain cyclization is the formation of an amide structure between an aspartic acid or glutamic acid residue and a basic amino acid, which requires the side chain protection of the polypeptide, whether on the resin or after dissociation. The base must be selectively removable. The third side chain-side chain cyclization is the formation of a diphenyl ether by tyrosine or p-hydroxyphenylglycine. This type of cyclization in natural products is only present in the microbial product, and the cyclized product tends to have potential pharmaceutical value. The preparation of these compounds requires unique reaction conditions and is therefore not commonly used in the synthesis of conventional polypeptides.
(2) terminal-to-side chain
Terminal-side chain cyclization typically involves the C-terminus with the amino group of the lysine or ornithine side chain, or the N-terminus with an aspartic acid or glutamic acid side chain. Still other polypeptide cyclizations are formed by the formation of an ether bond at the terminal C with a serine or threonine side chain.
(3) Terminal-terminal or head-to-tail
The chain polypeptide can be cyclized in a solvent or immobilized on a resin and cyclized by a side chain. Cyclization in a solvent should use a low concentration of the polypeptide to avoid oligomerization of the polypeptide. The yield of the head-to-tail synthesis of a cyclic polypeptide depends on the sequence of the chain polypeptide. Therefore, before large-scale preparation of cyclic polypeptides, a library of possible stranded lead polypeptides should first be created and then circularized to find sequences that yield the best results.
2, N-methylation
N-methylation is first introduced in natural polypeptides and introduced into the synthesis of peptides to prevent the formation of hydrogen bonds, thereby making the polypeptide more resistant to biodegradation and clearance. The synthesis of polypeptides using N-methylated amino acid derivatives is the most important method. Alternatively, the Mitsunobu reaction can be carried out with N-(2-nitrobenzenesulfonyl chloride) polypeptide-resin intermediate and methanol. This method has been used. A library of cyclic polypeptides containing N-methylated amino acids is prepared.
3. Phosphorylation phosphorylation is one of the most common post-translational modifications in nature. In human cells, more than 30% of proteins are phosphorylated. Phosphorylation, especially reversible phosphorylation, plays an important role in the control of many cellular processes, such as signal transduction, gene expression, cell cycle and cytoskeletal regulation, and apoptosis.
Phosphorylation can be observed on a variety of amino acid residues, but the most common phosphorylation targets are serine, threonine and tyrosine residues [4]. Phosphotyrosine, phosphothreonine and phosphoserine derivatives can be introduced into the polypeptide either in the synthesis or after the synthesis of the polypeptide. Selective phosphorylation can be achieved using serine, threonine and tyrosine residues that selectively remove the protecting group. Some phosphorylating agents can also introduce a phosphate group into the polypeptide by post-modification [5]. In recent years, some scholars have used the chemically selective Staudinger-phosphite reaction to achieve site-specific phosphorylation of lysine (Figure 3).
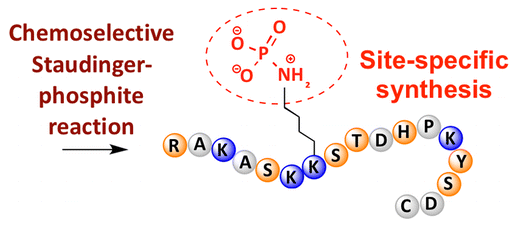
4. Myristylation and Palmitoylation The acylation of the N-terminus with a fatty acid allows the polypeptide or protein to bind to the cell membrane. The N-terminally myristoylated sequence allows the Src family of protein kinases and the reverse transcriptase Gaq protein to be targeted to bind to cell membranes. Myristate can be attached to the N-terminus of the resin-polypeptide using standard coupling reactions, and the resulting lipopeptide can be dissociated under standard conditions and purified by RP-HPLC.
5. Glycosylated glycopeptides such as vancomycin and teicoplanin are important antibiotics for the treatment of drug-resistant bacterial infections, and other glycopeptides are often used to stimulate the immune system. In addition, since many microbial antigens are glycosylated, it is important to study the glycopeptides to improve the therapeutic effect of infection. On the other hand, studies have found that proteins on the cell membrane of tumor cells exhibit abnormal glycosylation, which makes glycopeptides also play an important role in cancer and tumor immune defense research. The preparation of glycopeptides generally utilizes the Fmoc/t-Bu method. Glycosylation residues, such as threonine and serine, are often introduced into the polypeptide via a pentafluorophenol ester-activated Fmoc protected glycosylated amino acid.
6. Prenylation of prenylation occurs at the cysteine ​​residue on the side chain near the C-terminus. Prenylation of proteins can increase cell membrane affinity and form protein-protein interactions. The prenylated proteins include tyrosine phosphatase, small GTPase, synergistic chaperone molecules, nuclear lamina and centromeric binding proteins. The prenylated polypeptide can be prepared by a prenylation method on a resin or by introducing a cysteine ​​derivative [9].
7, polyethylene glycol (PEG) modification
PEG modification can be used to improve proteolytic stability, biodistribution, and peptide solubility [10]. The introduction of PEG chains on the polypeptides can improve their pharmacological properties and also inhibit the hydrolysis of the polypeptide by proteolytic enzymes. PEG polypeptides pass the glomerular capillary section more easily than ordinary polypeptides, greatly reducing renal clearance. Since the effective half-life of PEG polypeptides is prolonged in vivo, normal therapeutic levels can be maintained using lower dose, lower frequency polypeptide drugs. However, PEG modification also has a negative effect. A large amount of PEG also prevents the enzyme from degrading the polypeptide while also reducing the binding of the polypeptide to the target receptor. However, the low affinity of the PEG polypeptide is usually offset by its longer pharmacokinetic half-life, and by being present in vivo for a longer period of time, the PEG polypeptide has a greater likelihood of being absorbed by the target tissue. Therefore, the specifications of PEG polymers should be optimized for optimal results. On the other hand, due to a decrease in renal clearance, PEG polypeptides accumulate in the liver causing macromolecular syndrome. Therefore, PEG modification needs to be designed more carefully when the peptide is used for drug testing.
Common modifying groups for PEG modifiers can be summarized as follows: amino (-Amine)-NH2, aminomethyl-CH2-NH2, hydroxy-OH, carboxy-COOH, thiol-(Thiol)-SH, maleimide -MAL, succinimide carbonate-SC, succinimide acetate-SCM, succinimide propionate-SPA, N-hydroxysuccinimide-NHS, propionate-CH2CH2COOH, aldehyde group -CHO (eg propionaldehyde-ALD, butyraldehyde-butyrALD), acrylic acid (-Acrylate)-ACRL, azido-Azide, biotinyl-Biotin, fluorescein-Fluorescein, glutaric acid-GA, acyl Mercapto-Hydrazide, alkynyl-Alkyne, p-toluenesulfonate-OTs, succinimide succinate-SS, and the like. A PEG derivative bearing a carboxylic acid can be coupled to an N-terminal amine or lysine side chain. The amino-activated PEG can be coupled to an aspartic acid or glutamic acid side chain. MAL-activated PEG can be coupled to a fully deprotected cysteine ​​side chain thiol [11]. The PEG modifiers are generally classified as follows (Note: mPEG ie methoxy-PEG, CH3O-(CH2CH2O)n-CH2CH2-OH):
(1) Linear PEG modifier
mPEG-SC, mPEG-SCM, mPEG-SPA, mPEG-OTs, mPEG-SH, mPEG-ALD, mPEG-butyrALD, mPEG-SS
(2) Bifunctional PEG modifier
HCOO-PEG-COOH, NH2-PEG-NH2, OH-PEG-COOH, OH-PEG-NH2, HCl·NH2-PEG-COOH, MAL-PEG-NHS
(3) Branched PEG modifier
(mPEG)2-NHS, (mPEG)2-ALD, (mPEG)2-NH2, (mPEG)2-MAL
8. Biotinylated biotin can bind strongly to avidin or streptavidin, and the binding strength is even close to the covalent bond. Biotinylated peptides are commonly used in immunoassays, tissue cytochemistry and fluorescence-based flow cytometry. Labeled avidin antibodies can also be used to bind biotinylated polypeptides. The biotin label is often attached to the lysine side chain or to the N-terminus. Usually, 6-aminocaproic acid is used as a link between the polypeptide and the biotin, and the bond can bind the substrate flexibly and can bind better in the case of steric hindrance.
9. Fluorescently labeled fluorescent labels can be used to track peptides in living cells, as well as to study enzymes and mechanisms of action. Tryptophan (Trp) is fluorescent and can therefore be used for intrinsic labeling. The emission spectrum of tryptophan depends on the external environment and decreases as the polarity of the solvent decreases. This property is useful for detecting polypeptide structure and receptor binding [12]. Tryptophan fluorescence can be quenched by protonated aspartic acid and glutamic acid, which may limit its use. The dansyl chloride group (Dansyl) is highly fluorescent when bound to an amino group and is also commonly used for fluorescent labeling of amino acids or proteins.
Fluorescence resonance energy conversion (FRET) is very useful for the study of enzymes. When FRET is used, the substrate polypeptide often contains a fluorescent labeling group and a fluorescence quenching group. The labeled fluorophore will be quenched by the quencher by non-photon energy transfer. When the polypeptide is dissociated from the enzyme under investigation, the labeling group emits fluorescence.
10. The cage polypeptide polypeptide has an optically removable protecting group, and the optically removable protecting group can block the binding of the polypeptide to the receptor. When exposed to UV, the polypeptide is activated to restore affinity to the receptor. Since this optical activation can be controlled by time, amplitude or position, clathra polypeptides can be used to study reactions occurring within cells [13]. The protecting groups most commonly used for cage polypeptides are 2-nitrobenzyl and derivatives thereof which can be introduced in the synthesis of polypeptides by protected amino acid derivatives. Amino acid derivatives which have been developed are lysine, cysteine, serine and tyrosine. Aspartic acid and glutamic acid derivatives are not commonly used because they are easily cyclized during peptide synthesis and dissociation.
11. Polypeptide (MAP)
Short peptides are generally not immunogenic and must be coupled to a carrier protein to produce antibodies. Polypeptide (MAP) consists of multiple identical polypeptides linked to a lysine nucleus that specifically express highly efficient immunogens and can be used to prepare peptide-carrier protein couplers. The MAP polypeptide can be synthesized stepwise on a MAP resin by solid phase synthesis. However, incomplete coupling can result in the loss or truncation of peptide chains on some branches and thus does not exhibit the properties of the original MAP polypeptide. As an alternative method, the polypeptide can be prepared and purified separately and then coupled to the MAP [14]. The sequence of the polypeptide linked to the core of the polypeptide is well defined and readily characterized by mass spectrometry.
Conclusion:
Polypeptide modification is an important means of designing polypeptides. Chemically modified peptides can not only maintain high biological activity, but also effectively avoid the disadvantages of immunogenicity and toxicity. At the same time, chemical modification can give the peptide some new excellent properties. . In recent years, the method of post-modification of peptides by means of CH activation has also been rapidly developed, and many important achievements have been made. Later, the author will also review this field, so stay tuned!
Universal A.I. Sheaths With Blue Insert
Blue Insert Universal A.I. Sheaths,Universal Sheaths,Sheath Blue Insert Split-Non,Endoscope Hydrophilic Coating Dilators
Jinan Mucho Commercial Inc. , https://www.muchovet.com